Decompression Stress: Post-Dive Fatigue and Subclinical DCS
Most divers know about decompression sickness. But have you heard about decompression STRESS?
The effect of nitrogen bubbles on our bodies is far more complex and nuanced than most diving manuals describe. It is not a black-and-white issue where the diver is either perfectly fine or blatantly symptomatic.
Inert gas micro-bubbles exist within divers following every ascent. However, they may not grow large enough to be recognized as a painful medical problem. Nonetheless, they can still have a profound effect on the diver.
The prevalence of post-dive fatigue
Post-dive fatigue, malaise, lethargy, sleepiness, and decreased vitality are often-observed consequences of scuba diving. Many divers can relate to this; having felt sluggish and in dire need of a snooze during surface intervals or on the boat ride back to shore.
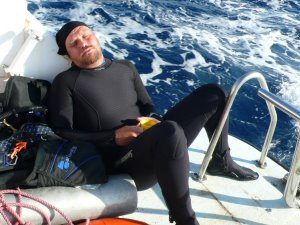
- Is it really normal and expected?
- Is it decompression stress?
- Is it significant of a hyperbaric physiological impact on our body?
- Is it harmful to our health and well-being?
- Can it be avoided?
Inexplicable tiredness after diving may be sub-clinical DCS
There are medical/scientific studies potentially indicating that decompression stress resulting from increased microbubble quantity can be a significant factor in the development of sub-clinical decompression sickness (DCS). This occurs through the activation of the body’s immune system response.
This sub-clinical DCS results from complex changes in blood/brain chemistry and might manifest as unusual post-dive fatigue or reduced vitality in divers. There is an increasing concern that long-term health consequences may occur due to repeated occurrence of decompression stress.
A Personal Account of Decompression Stress
Over thousands of dives and several decades of diving, I have noticed a vivid change in my expectations of post-dive fatigue.
As a novice diver, I used to feel it was normal to be sleepy and listless after diving. I dismissed it as a result of being colder, being in the water, having too much fresh air, and more exertion than normal. But it wasn’t. There was an element of denial in those explanations.
The signs of decompression stress
Working as a recreational diving instructor in Thailand; I invariably slept on the dive boat between dives and fell asleep on the couch for an hour or two when I got home from diving. The urge to sleep was irresistible.
For the first time in my life, I started suffering severe, systemic allergic reactions but found no cause. I was physically fit, well rested, well fed; and very acclimatized to the level of activity, diving routine, and water conditions. Diving was a daily routine.
The dives were not technically challenging, they were entry-level training, but they involved multiple ascents, controlled emergency swimming ascent (CESA) practice, and shepherding students yet to master their buoyancy control.
It was hard work, but no certainly less challenging than the constant physical exertion I had become adapted to when I previously served in the military. It became increasingly implausible to deny that my post-dive fatigue was unnatural.
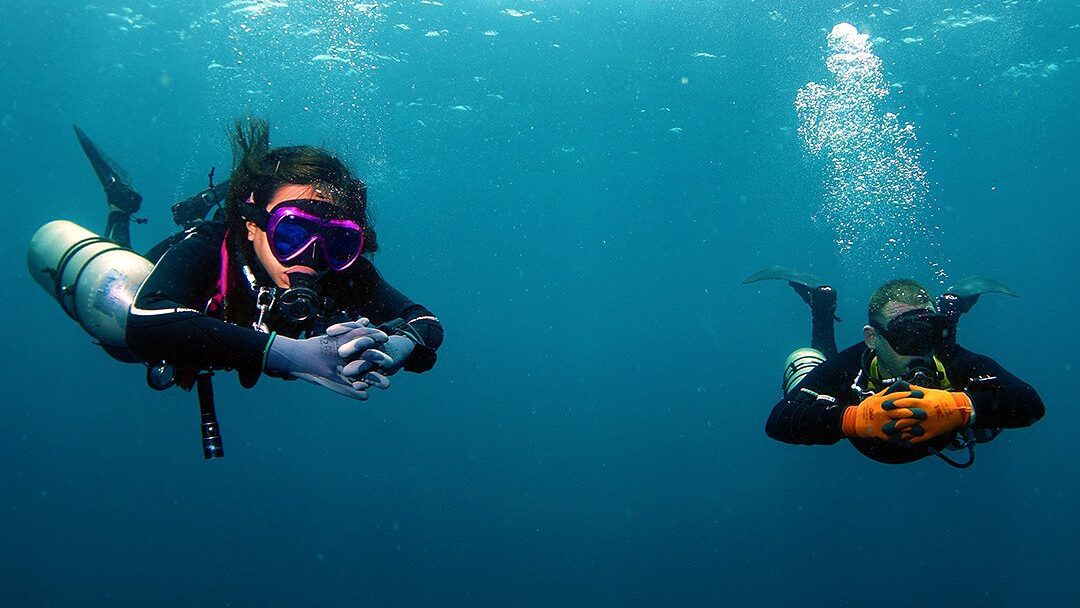
Learning about decompresion stress as a technical diving instructor
Over the last two decades, my diving became primarily technical in nature. I work as a technical diving instructor, routinely completing deep decompression dives.
It quickly became apparent to me that, even though I was conducting dives with a much higher degree of saturation, my post-dive vitality had returned to a high level. I dove several mixed-gas decompression dives a day but still felt invigorated and energetic afterward.
My technical dives were longer, colder, and much more physically demanding than my prior recreational teaching dives, but I no longer suffered fatigue as a result.
Experimenting to reduce decompression stress
I experimented with different decompression algorithms, ascent profiles, and gradient factors.
My feedback was paying close attention to my level of post-dive energy, wakefulness, and sense of well-being.
Through this self-assessment, in conjunction with undertaking a range of decompression strategies, I could determine and optimize the effectiveness of my off-gassing and microbubble management.
I had become educated about subclinical DCS and the term ‘decompression stress’. It was now impossible for me to accept post-dive fatigue as a normal, natural, or unavoidable consequence of diving.
Become A Shearwater Power User! (eBook)
An Illustrated Guide to Gradient Factors and Advanced Shearwater Computer Settings for safe scuba diving.
42 Pages. Printable PDF format. Fully Illustrated. $9
Become A Shearwater Power User eBook
As featured on the DiveTalk Podcast
Decompression Sickness (DCS) Types
Decompression sickness is caused by inert gases leaving solution inside the body and forming bubbles. If allowed to grow sufficiently large, those bubbles present signs and symptoms, which allow DCS to be diagnosed, such as;
DCS Type 1: Minor
Localized pain, skin itching (Pruritis) and skin rash (Cutis marmorata)
DCS Type 2: Cardiovascular/Pulmonary and Neurological
Severe pain, organ failure, decreasing levels of consciousness, cognitive ability, communication, mobility, dexterity, sensation and bodily control (including; breathing, bladder and bowel control).
DCS Type 3: Vestibular
Inner-Ear bubble, generally due to Isobaric Counter-Diffusion when using Trimix for dives below 100m depth. It presents as severe vertigo, nausea, and localized ear/head pain.
DCS Type 4: Osteopathic
Dysbaric Osteo-Necrosis (DON) is a long-term condition where frequent and repeated exposure to hyperbaric conditions (increased pressure) and variation (pressure changes). In turn, it causes necrosis (death/brittleness) of the skeletal bones.
Beyond osteopathic symptoms, there is an increasing medical discussion on other long-term subclinical issues. These include:
- Brain lesions
- Central nervous system damage
- Eye degradation
- Damage to other organs
Whilst these effects have been noted in studies, they are not yet proven. However, they may be added to the list in the future and I anticipate that we might see further classifications of DCS in the following years as further research is conducted.
We can see that the severity and type of DCS diagnosis is related to the frequency (quantity), location, and most importantly, size of the bubbles that occur in the body.
However, DCS is only diagnosed if it conforms with the symptoms known for the types listed above; and if it reaches a level of severity that provokes the victim to seek medical attention.
Subclinical DCS
DCS is not an all-or-nothing ailment. It is a scale of severity. On that scale, there is a point below which the victim is not at risk of immediate physical harm. The symptoms may be too subtle for diagnosis and/or there is no proven need for treatment.
The Definition of Subclinical
- Relating to or denoting a disease that is not severe enough to present definite or readily observable symptoms.
- Of disease or injury, without signs and symptoms that are detectable by physical examination or laboratory test; not clinically manifest.
- In diagnosis, where some criteria are met but not enough to achieve the clinical status
Bubbles occur in our bodies on the ascent from most scuba dives, but those bubbles have to reach a sufficient size before they cause enough immediate harm to present symptoms that allow a diagnosis and treatment of DCS.
Subclinical DCS: Bubbles, but small ones
Bubbles of insufficient size to cause clinically identifiable decompression sickness symptoms are named ‘micro-bubbles’. These bubbles may remain subclinically small in size but may occur in large numbers.
They may also, in sufficiently large numbers, still have a noticeable (if not immediately harmful) impact on us.
It has been proposed that post-dive unnatural fatigue, malaise, reduced vitality, and drowsiness are symptoms of many tiny bubbles. This is called “subclinical”, ‘non-symptomatic’ or “mild” DCS. Historically, it has been considered harmless.
“… “mild DCI” is harder to define. This is largely because bubbles form, and can be detected in the venous blood, following a significant proportion of dives that do not result in symptoms that would normally be labelled “DCI”. It is therefore hardly surprising that there can be a poorly defined boundary between wellness and mild DCI following diving. […] It is not infrequent for divers to report highly non-specific and vague symptoms of variable latency, such as mild evanescent aches and pains, fatigue, demotivation and headache”.
Management of Mild or Marginal Decompression Illness in Remote Locations. S.J.Mitchell Ph.D, D. J. Doolette, Ph.D. DAN Remote Workshop Proceedings 2005
“Inappropriate fatigue is a clear-cut symptom of subclinical decompression illness. I’m not a technical diver, Lord knows; but I see a lot of these guys. I send them out with decompression tables and they tell me what happens. They use their fatigue, their feeling of well-being – we called it constitution yesterday – as a major item in judging whether their decompression was adequate. The fact that they don’t have any joint pain or tingling or rash or anything is secondary. Their major thermometer as to whether they’ve been properly decompressed is how well they feel. And that has to do with the fatigue and inappropriate fatigue. So we have to keep this as a symptom, not as a side issue”.
Management of Mild or Marginal Decompression Illness in Remote Locations. S.J.Mitchell Ph.D, D. J. Doolette, Ph.D. DAN Remote Workshop Proceedings 2005
“Doppler monitoring has revealed the presence of gas phase bubbles in divers ascending even from relatively shallow dives. We have also all experienced symptoms such as headache and fatigue in the immediate post-dive phase which we have put down to overexertion on exiting the water etc. In more recent years, we have been told that these symptoms are those of subclinical DCI and we have lived with these, perhaps naively, thinking that because they are not associated with overt symptoms of DCI, they are not causing any damage and are nothing to worry about. However, enter the iceberg principle. Nine tenths of the damage may be invisible and cumulative”.
The Trouble With Bubbles. Richard Heads PhD. 9-90 Magazine: UK Diving in Depth
Decompression stress as a sign of subclinical DCS
Decompression stress is defined as the amount of inert gas dissolved in various tissues throughout the body (Alert Diver Magazine, Ascent Rates).
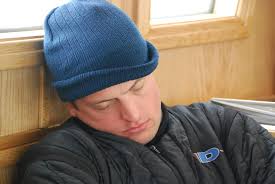
It is measured by counting ‘bubble scores’ (via the Spencer Scale, Powell Grade or KM code) on the size and frequency of micro-bubbles in the body after a dive using Doppler ultrasound devices.
“…we know that bubbles are probably present after most dives, so we don’t have to exceed an M-value to produce bubbles in the absence of DCS. […] Although an M-value may not have been exceeded, symptoms of decompression stress such as fatigue, malaise, drowsiness etc. could well be the result”.
The Trouble With Bubbles. Richard Heads PhD. 9-90 Magazine: UK Diving in Depth
“Someday, research might result in tests for physiological decompression stress when DCS symptoms are not apparent…”
Rethinking the Hit. Zaferes A. Immersed Spring 1997
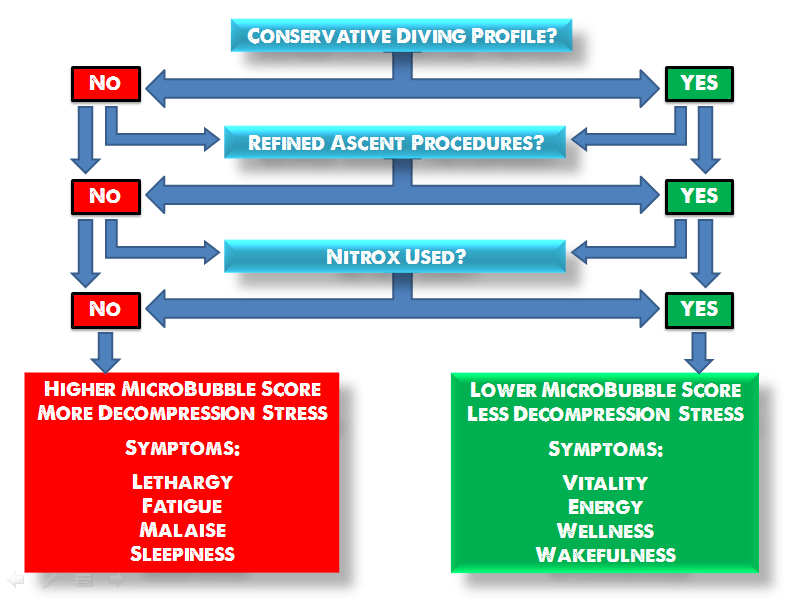
Micro-bubbles and subclinical DCS
It is well proven in multiple doppler ultrasound studies that virtually all divers develop small bubbles in their bodies during ascent. A quantity of these bubbles will persist during the surface decompression phase; determined by the effectiveness of in-water decompression.
However, these micro-bubbles (also known as silent bubbles) are insufficiently large to cause us significant, immediate physical harm. The frequency (number) of those bubbles is determined by the effectiveness of off-gassing nitrogen on the ascent. Poor off-gassing leads to ‘decompression stress’ and is represented by a larger frequency of micro-bubbles in the body.
Decompression models used in dive tables and computers assign a maximum value (m-value) to ascent supersaturation in order to prevent those micro-bubbles from growing to a size that causes clinically diagnoseable harm (symptomatic decompression sickness).
Bubble model algorithms care about bubble size
Some modern decompression models, known as ‘bubble models’, also include calculations that reduce the number of those micro-bubbles by off-gassing vulnerable fast tissues at deeper depths; as an additional precaution against DCS. The general principle is that if the quantity of micro-bubbles (also known as bubble ‘seeds’) is reduced early in the decompression phase, there is less potential for anything to grow into DCS symptomatic bubbles at a later stage.
Decompression models stop large bubbles, not small ones
Nonetheless, all modern decompression models are concerned with preventing bubbles from growing to a harmful size. However, they are not concerned about limiting the frequency of smaller bubbles. This keeps you out of a decompression chamber, but neglects the impact on post-dive vitality and long-term health.
Decompression calculations are always a trade-off between safety and time. Until diving medicine can prove any long-term health implications of decompression stress, that is likely to continue.
Factors known to influence off-gassing efficiency and micro-bubble elimination include; ascent speed, ascent stops, dive profile, repetitive dives, bounce dives and ascent gas selection.
Decompression stress: Ascent speed
The ascent speed dictates the relationship between tissue super-saturation and the rate at which nitrogen can be eliminated by the body. Excessive speed causes nitrogen to form bubbles which can grow to a DCS symptomatic size. Insufficient speed limits off-gassing efficiency by lowering the pressure gradient between saturated nitrogen and the surrounding atmosphere (the difference between the partial pressure of nitrogen absorbed within the body and that within the gas we are breathing).
For more details on ascent speed and micro-bubble formation, read; ‘Best Ascent Speed For Scuba Diving‘.
Decompression stress: Ascent stops
Pausing on the ascent at specific depths allows nitrogen to be eliminated from the body; both saturated nitrogen from the tissues and by shrinking or eliminating nitrogen micro-bubbles. This was proven by Donna M Uguccioni in her paper, ‘Doppler Detection of Silent Venous Gas Emboli in Non-Decompression Diving Involving Safety Stops’:
“Dives with safety stops showed significantly fewer venous gas emboli. Other factors, such as, depth and bottom time influenced the efficiency of safety stops. Overall, safety stops are capable of significantly reducing venous gas emboli detected by Doppler in the blood of human divers”.
Uguccioni’s study proved not only that a safety stop was beneficial to reducing the frequency of micro-bubbles, but also that multiple stops at 9, 6, and 3m depth were more beneficial than a single stop at 5m.
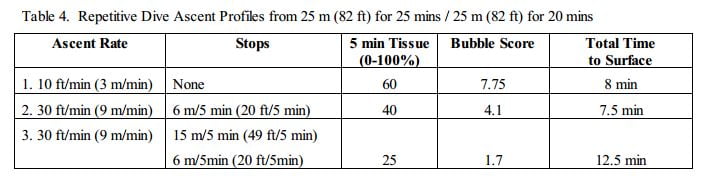
Decompression stress: Dive profile
The profile of individual dives or repetitive or multi-day diving routines is an important factor in determining bubble formation. Saw-tooth dive profiles create more bubbles with each rise in depth. This cumulative effect leads to a much higher bubble score by the time the final ascent is completed.
A similar situation occurs over a longer time period when divers complete intensive repetitive diving (3+ dives per day) and extended periods of multi-day diving. This is common behavior on diving-focused vacations or for novice divers with weak buoyancy control who struggle to maintain consistent depth on shallow dives.
The Trouble With Bubbles. Richard Heads PhD. 9-90 Magazine: UK Diving in Depth
“…one reason why sawtooth profiles are bad, because the bubbles formed on each ascent phase are not crushed again but accumulate, such that the final ascent and decompression phase is started with a pre-existing bubble load leading to a provocative situation. Since it is now recognized that the lifetime of a bubble can be measured in days, this has implications for repetitive and multi-day diving, in particular”.
Decompression stress and ascent gas selection
Utilizing an appropriate ascent gas breathing mix reduces tissue tension across the surface of micro-bubbles, helping to maintain a negative pressure gradient that promotes effective off-gassing and bubble elimination. The higher the percentage of oxygen, the more efficient the pressure gradient is to shrink bubbles and remove nitrogen from the body.
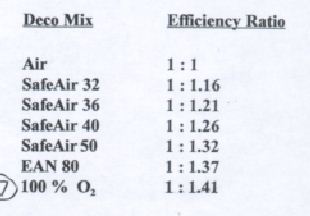
“In layman’s terms, venous microbubbles screw up the gas exchange (ie. inert gas elimination) in the lungs, an effect which can be completely eliminated by decompressing on oxygen. So let’s face it if oxygen recompression therapy is the treatment of choice for DCS, why not treat ourselves in situ to prevent subclinical DCS or decompression stress ?”
The Trouble With Bubbles. Richard Heads PhD. 9-90 Magazine: UK Diving in Depth
Adhering to a proper ascent speed, multiple shallow safety stops, and ideal ascent gasses are not taught within the curriculum of many recreational diving agencies.
In short, currently taught recreational diving practices may not offer sufficient minimization of decompression stress, especially when repetitive and multi-day shallow diving occurs.
“What I find alarming, is that some entry level training agencies (not to mention any names), given all this recent knowledge and changing trends, haven’t altered their tables since their inception. The question is whether as instructors we should alter our methods to take some of these things into account. I think that certainly as experienced divers we should adopt safer decompression strategies. The recommendations for entry level training are obviously more limited, but I think we have a responsibility to adopt the safest possible methods for our trainees given the knowledge available.”
The Trouble With Bubbles. Richard Heads PhD. 9-90 Magazine: UK Diving in Depth
Nitrox: Nitrogen absorption and release
Nitrox or Enriched Air Nitrox (EANx) are scuba diving gas mixtures that contain a higher than normal (>21%) content of oxygen.
These mixtures consequently contain a proportionally smaller amount of nitrogen (<79%), so divers use nitrox to reduce the amount and/or rate of nitrogen absorption during a dive. This allows them to dive for a longer time at a given depth (increased No-Stop limit), or to absorb less nitrogen on a given dive (depth + time) than if they breathed regular air.
The additional, but often not educated, benefit of nitrox is that the reduced partial pressure of nitrogen, coupled with increased partial pressure of oxygen, serves to increase the gas differential between tissues, lungs and ambient pressure.
This increased gas differential accelerates the rate of nitrogen release from our bodies on the ascent, compared to air, without allowing bubbles to grow.
The aim is always to off-gass
Simply put, we off-gas more efficiently as nitrogen partial pressure decreases. This is the principle that leads technical divers to switch gas to higher % oxygen deco mixes as they ascend from deep dives.
The practice is known as ‘accelerated decompression’; it serves to reduce the divers’ overall ascent time by maximizing the efficiency of off-gassing.
There has been fervent discussion amongst the diving community for decades on whether the use of nitrox reduces diving fatigue and/or promotes a feeling of post-dive wellness and vitality.
There are a great many divers who claim to feel noticeably better after diving if they use nitrox rather than air.
The number of these anecdotal accounts might give plausibility to a theory that nitrox reduced post-dive fatigue were it not for scientific testing which proved no such direct link exists.
Nitrox Studies And Post-Dive Fatigue
The anecdotal belief that nitrox, through some inherent property of the increased oxygen partial pressure in the body, reduced post-dive fatigue prompted several scientific studies.
These studies sought to determine whether any property of nitrox (for example, increased oxygenation of body tissues and/or brain) had a direct effect on the individual to reduce fatigue after diving.
The overall conclusion was that it did not.
“Despite a common impression that diving on nitrox may cause less fatigue than performing the same dive using an air mixture, scientific research to date has not found solid evidence to support such an assumption.”
Air, Nitrox and Fatigue. Nochetto M., M.D. Alert Diver Magazine. 2014
“Diving to 18m while breathing air produced no measurable difference in fatigue, attention levels, ability to concentrate or DHS scores, compared with breathing EANx 36%.”
Measurement of fatigue following 18 msw dry chamber dives breathing air or enriched air nitrox.Harris RJ, Doolette DJ, Wilkinson DC, Williams DJ (2003). Undersea and Hyperbaric Medicine (Undersea and Hyperbaric Medical Society) 30 (4): 285–91.
“Results from this study did not support the contention that using EAN36 as a breathing gas reduces post-dive fatigue. To date, research has indicated that there is no difference in fatigue levels between air and EAN36 dives.”
Measurement of Fatigue following 18 msw Open Water Dives Breathing Air or EAN36. Chapman SD, Plato PA. In: Brueggeman P, Pollock NW, eds. Diving for Science 2008. Proceedings of the American Academy of Underwater Sciences 27th Symposium.
However, these studies did not utilize dive parameters likely to bring about decompression stress. If there was no decompression stress, then nitrox had no impact on reducing fatigue that may result from it. To support that hypothesis, the study findings did note that in tests using nitrox, participants felt more healthy; which possibly indicated that lower decompression stress was present.
So, whilst there is no property inherently within nitrox or oxygen that directly reduces fatigue, it does promote more efficient off-gassing and reduce decompression stress on the ascent. It reduces the number and size of micro-bubbles in the body after a dive.
Where previous studies have examined the effect of nitrox on post-dive fatigue, their testing was conducted using identical dive profiles; which caused little or no decompression stress, with and without nitrox.
Thus, no impact of post-dive fatigue was noted.
If a comparison study was conducted on a range of dives calculated to exert low, medium, and high levels of decompression stress, then we might predict that nitrox would have a more identifiable impact on results indicating varying levels of wellness, energy, and vitality.
Micro-bubbles, DCS, and the immune system
It is known that bubbles within the body trigger an immune system ‘foreign body’ response. For this reason, immune-suppressant and anti-inflammatory drugs are often administered during hyperbaric treatment for DCS.
“Long thought to be a simple process of blockage of vessels and infarction, it has now been shown that there is a tripping of the complement system and the immune system (T- and B- leucocytes) when bubbles form from decompression. This immune response has a domino effect on blood chemistry that leads to marked changes in the tissues long after the bubbles are gone […] This same process might be the explanation of the excessive fatigue that many divers describe after diving – the fatigue actually being the complement activation damage that is know to occur distant from local bubble sites and the hemoconcentration that occurs.”.
The Complement System and Diving. Dr. Ernest Campbell FACS
“As the bubbles course through our body, an inflammatory signal is activated, and the nitrogen bubbles are easily trapped in the pathways through which they travel. The result is that blood flow is blocked–potentially harming vital organs such as the spinal cord and the brain. One possibility of decompression sickness risk reduction, as explored by the NMRC, involves blocking the immune system’s inflammatory response to intravascular bubbles with the use of anti-inflammatory drugs. This technique is regarded as one of the safest and most effective ways of avoiding decompression sickness”.
New Methods to Avoid Decompression Sickness. K Mogentale. ScientificAmerican.com
How the immune system responds to bubbles
The immune system reacts to bubbles as it would to any foreign body; such as a virus or bacteria. That reaction is as follows:
- When bubbles occur in the body they will attract blood proteins (complement system activation), as those proteins contact with bubbles, they change in molecular shape and solubility (denaturation).
- The denatured protein is recognized by the body and the body diverts white blood cells to ‘attack’ the bubble, whilst also releasing histamines to dilate blood vessels in the area (local inflammation) to aid the travel of the white blood cells to where they are needed.
- The release of histamine also causes the surrounding blood vessel lining to become sticky so that white blood cells surrounding the bubble will ‘stick’ in place and trap the bubble. This process is known as margination.
- The process of margination also causes cells in the blood vessel wall (endothelium cells) to separate and allow fluids, white blood cells and the bubble/s (if not too large) to leave the bloodstream and enter the surrounding tissues. This not only thickens the blood (hemoconcentration), but a sufficient loss of fluid from the bloodstream into the tissues can cause shock.
- The separation of endothelium cells causes an additional reaction in the body; as it triggers a release of platelets into the area. These platelets combine with collagen to form a clot in the tissue around the bubble. Platelets also release serotonin which counters the effect of histamine and constricts blood flow to the area.
When the bubble is sufficiently large, this process is intense and noticeable locally in the body; becoming symptoms of DCS.
Numerous occurrences of smaller bubbles will not have a local, obviously symptomatic, effect; but may have a lower-grade systemic impact. Whilst the immune system ‘ foreign body’ response can be effective in dealing with many maladies, it is profoundly counter-effective in dealing with bubbles.
“Decompression sickness affecting the nervous system is still a serious problem in diving, but the mechanisms involved are in dispute. Although microbubbles can be detected in the pulmonary artery on decompression using ultrasound, mammalian lungs are competent filters for microbubbles larger than 20 microns in diameter. It has been assumed that smaller bubbles released by the lungs are harmless, because there is evidence that they do not arrest in the cerebral circulation. […] experiments demonstrate that microbubbles impair the blood-brain barrier integrity to protein, causing focal edema”.
Microbubble Damage to the Blood-Brain Barrier: Relevance to Decompression Sickness. Hills BA, James PB. Undersea Biomed Res. 1991 Mar;18(2):111-6.
“…the loss of plasma from capillaries may significantly increase hemoconcentration and shock following DCS. This, in a less serious form, is probably the cause of “inappropriate fatigue,” a symptom of DCS”.
Rethinking the Hit. Zaferes A. Immersed, Spring 1997
“Bubbles are often found in tissues during gas-forming infections and in infection-prone states. In these diseases, intravascular gas offers a signal of infection to immune cells. Platelet activation by gas may often accompany a beneficial immune response to pathogens. Pathologic bubble-platelet interaction in decompression illness may be an example of gene-environment mismatch.
Gene-environment mismatch in decompression sickness and air embolism. Alcock J, Brainard AH. Med Hypotheses. 2010 Aug;75(2):199-203. doi: 10.1016/j.mehy.2010.02.022. Epub 2010 Mar 19.
[…] The spectrum of DCI symptomatology extends from simple malaise to full cardiovascular collapse. Central nervous system (CNS) injury may be subtle or blatant and peripheral nerves may be involved. DCI may also present as a variant of the systemic inflammatory response syndrome”.
If the bubbles are small in size, this immune system ‘foreign body’ response may remain symptomatically unnoticeable to the victim. But if the occurrence of micro-bubbles is, nonetheless, high in frequency, then there may be widespread low-intensity immune reactions throughout the body. A subclinical reaction, but a reaction that does have a significant impact on the individual’s well-being.
That impact may present as general fatigue, weariness, sleepiness, listlessness, reduced vitality, decreased energy, somnolence; or just a sense of being under-par physically or mentally.
Seratonin and CNS Fatigue
Central Nervous System Fatigue (also known as “Central Fatigue”) is neurochemical fatigue triggered by changes in the function of the central nervous system, not explained by dysfunction of the muscle. It is typically brought about by endurance exercise which may cause consequent neurochemical changes in the brain; primarily the increased release of serotonin (5HT).
Whilst there are no current scientific tests to prove a direct causal link between the neurochemical changes and fatigue, existing methods have provided enough evidence to suggest that serotonin and dopamine are significant drivers of central nervous system fatigue.
“Serotonin has been linked to fatigue because of its well known effects on sleep, lethargy and drowsiness and loss of motivation.”
Central fatigue: the serotonin hypothesis and beyond” Meeusen R1, Watson P, Hasegawa H, Roelands B, Piacentini MF. Sports Med. 2006;36(10):881-909.
We have seen that serotonin can be induced as a vasodilator during the immune system reaction to bubble occurrence in the body, so much so that anti-inflammatory drugs are now the norm in treating DCS. However, serotonin’s primary role in the body is to act as a neurotransmitter in the central nervous system. Within the central nervous system, it regulates arousal, behaviour, sleep, and mood.
In sports science, is known as the central nervous system’s means of transmitting the symptoms of fatigue; which alerts athletes as to when physiological conditions are not tolerable. Thus, it provokes a need to rest and/or refuel during prolonged exercise. It seems possible to hypothesise that the existence of decompression stress through the micro-bubble formation in the body may also induce this neurochemical demand, which could present as short-term CNS fatigue.
“Good evidence suggests that increases and decreases in brain 5-HT (serotonin) activity during prolonged exercise hasten and delay fatigue, respectively…”
Possible mechanisms of central nervous system fatigue during exercise. Davis JM1, Bailey SP. Med Sci Sports Exerc. 1997 Jan;29(1):45-57.
Microbubbles and Brain Damage
We have seen that decompression stress, and certainly, decompression sickness, have an impact on the immune system. There is also an increasing concern that microbubbles can cause damage to the brain; in particular ischemic lesions and focal oedema.
As recently as 1989, the issue of microbubbles affecting the brain was unproven:
“The supposition of any damage to the brain rests on the occurrence of so called silent bubbles occurring in the blood or brain and spinal cord. That such bubbles do exist has been well demonstrated by Doppler technology in blood and tissue studies of animals’ spinal cords. Whether or not, however, these silent bubbles are the cause of changes in the brain is unproved…”
Evidence For Cerebral Perfusion Deficits in 28 Cases Of Dysbarism. Adkisson GH, Macleod MA, Hodgson M, et al. Lancet 2:119-121, 1989
However, by 1991, the immune response to microbubbles was shown to influence blood-brain barrier permeability and cause swelling:
“Hills & James demonstrated in 1991, that micro DCS bubbles increase blood-brain barrier permeability, resulting in local swelling and possibly the cerebral features of DCS, changes in cerebral blood vessels and spinal cord myelin and axon damage”
Rethinking the Hit. Zaferes A. Immersed, Spring 1997 (citing ‘Microbubble Damage to the Blood-Brain Barrier: Relevance to Decompression Sickness‘. Hills B.A., James P.B. Undersea Bio – medical Research. 1991, 18, 2:111-116).
Then, in 2013, Martin Juenemann and his team demonstrated that bubble size may not determine the severity of brain damage. In failing to prove that larger bubbles caused larger infarctions, they actually may have shown that smaller bubbles can cause equal, if not more, damage in sufficient numbers. Large bubbles are theorized to split into multiple small bubbles as they pass through the brain’s vascular system. They may also be constricted by smaller blood vessels.
Smaller bubbles can pass more readily into the brain and, in number, present a higher surface area, thus increasing the interface between gas, liquid and the interior surface of blood/lymphatic vessels (endothelium). The degree of this interface is known to be a significant factor of cerebral air microembolization-mediated tissue damage, as it activates the inflammatory response, the complement and clotting systems.
“Due to the extensive increase of bubble surface with smaller bubbles and the suspected noxiousness of the gas–liquid-endothelium contact, we considered ischemic infarction to increase in a comparable order of magnitude. The size of ischemic lesions, as detected by the MRI scan, appeared to be almost doubled for small bubbles;
Impact of Bubble Size in a Rat Model of Cerebral Air Microembolization. Juenemann et al. Journal of Cardiothoracic Surgery 2013, 8:198
[…] In summary, we could not find any significant differences between the two bubble sizes concerning functional outcome parameters, number of infarctions, or lesion volume, despite a 45-fold increase in the number of bubbles […]
According to a dynamic bubble behavior, bubbles may not maintain their size and split, aggregate or dissolve during downstreaming within short time. This may lead to a uniform bubble size within the terminal vascular bed, causing comparable probability of infarction, lesion volume, and consecutive functional decline […] Eliminating bigger bubbles seems to be insufficient to prevent post-intervention deterioration […] furthermore, there is experimental evidence that even gas bubbles small enough to pass through arterioles and the capillaries without verifiably occluding the vessel can lead to neurological deficits”
It is not yet known what quantity of cerebral air microembolization (microbubbles), along with other factors, is necessary to cause significant problems. However, it is proven that microbubbles can lead to “persistent focal neurological and neuropsychological deficits, often without the display of manifest cerebral infarction on MRI”. Activation of the immune system response by microbubbles is to blame.
“Pathophysiological background, thresholds for emboli load, and other determinants of clinical outcome after embolization are largely unknown. Mechanisms of CAM-induced tissue damage exceed mere mechanical consequences such as compression against the endothelial capillary wall and obstruction of blood flow by a lodging bubble: Constituting a foreign substance, air bubbles provoke clotting activation, inflammatory response with neutrophil aggregation and complement activation] with the production of radical species leading, amongst others, to increased vascular permeability and triggering a complex immunological and ischemic cascade of tissue injury. These pathomechanisms are initiated on the surface of the bubble with the gas–liquid-endothelium interface as a driving force”.
Impact of Bubble Size in a Rat Model of Cerebral Air Microembolization. Juenemann et al. Journal of Cardiothoracic Surgery 2013, 8:198
Further research on microbubbles and cerebral arterial gas embolism will undoubtedly make the situation clearer. The link between the number of microbubbles and the severity of the damage, or the long-term effects of repetitive low-scale damage remains to be identified.
However, this research is likely to shape dive planning strategies dramatically; as it adds much more emphasis to the need for timely microbubble elimination and a reduction in acceptable decompression stress. As mentioned earlier, it could form the basis of a new ‘Type 5’ DCS classification.
Practical measures to avoid decompression stress
Suffering unusual fatigue after a dive is neither natural nor normal. It can be indicative of decompression stress which may result in harmful effects on your blood/brain chemistry. Remember that the long-term medical implications of repetitive exposure to decompression stress are not yet known, but studies are beginning to identify potential issues stemming from microbubbles activating the complement and immune systems.
From my (very limited) medical understanding, the frequency (quantity) of bubbles would have a much more direct impact on immune system response.
Therefore, sub-clinical DCS doesn’t present the size of bubbles needed to provoke the symptoms (pain, rash etc) that justify chamber rides; but the quantity of (micro) bubbles can still bring about harmful changes in blood/brain chemistry. The symptoms of these changes might be unusual post-dive fatigue, lethargy, sleepiness and decreased vitality.
Preventing the formation of microbubbles, or resolving them at the earliest possible opportunity, may have significant health impacts for divers. Nitrox is one of several important factors that can help reduce decompression stress and the complement/immune system activation that causes unusual fatigue.
Steps to minimize decompression stress
Steps that divers can take to minimize decompression stress are:
- Dive conservatively within no-stop limits
- Apply additional conservatism settings on your personal dive computer or planning software.
- Refine safety stop procedures: 1 min at 9m/30ft, 2 min at 6m/20ft, 3 min at 3m/10ft.
- Accurately apply optimal ascent rates: 9m/20ft per min until the 1st stop, 3m/10ft per min thereafter.
- Choose a decompression model/computer algorithm designed to reduce microbubble scores (i.e. VPM-B or Buhlmann GF)
- Utilize an appropriate EANx mix as your bottom gas.
- Utilize 50% O2 for optimal off-gassing on your ascent above 21m/70ft
- Never conduct saw-tooth or bounce dive profiles
- Limit multi-day diving to allow frequent non-diving days
- Take heed of your post-dive vitality and adjust your diving practices accordingly.
Footnote
The information contained in this article solely represents the opinions of the author and is by no means an exhaustive review of all the available literature on decompression stress and bubble-activated immune responses. Much of the information is derived from other more detailed articles and these are referenced throughout. The reader is urged to refer particularly to the articles referenced for more detailed handling of the subject material. As far as possible and within the limitations of the author’s knowledge, the information contained herein is
deemed correct. However, it is a personal interpretation and as such, may not wholly represent the opinions of everyone involved in this complex area of research.
References
- Management of Mild or Marginal Decompression Illness in Remote Locations: An Introduction to the Problem S.J.Mitchell Ph.D, D. J. Doolette, Ph.D. DAN Remote Workshop Proceedings 2005
- The Trouble With Bubbles. Richard Heads PhD. 9-90 Magazine: UK Diving in Depth
- Rethinking the Hit. Zaferes A. Immersed Spring 1997
- Doppler Detection of Silent Venous Gas Emboli in Non-Decompression Diving Involving Safety Stops. Uguccioni, DM. 1994
- What Ascent Profile for the Prevention of Decompression Illness? . Marroni A et al. DAN Europe Special Project 01-2002
- Air, Nitrox and Fatigue. Nochetto M., M.D. Alert Diver Magazine. 2014
- Measurement of fatigue following 18 msw dry chamber dives breathing air or enriched air nitrox. Harris RJ et al. Undersea and Hyperbaric Medicine (Undersea and Hyperbaric Medical Society) 30 (4): 285–91. 2003
- Measurement of Fatigue following 18 msw Open Water Dives Breathing Air or EAN36. Chapman SD, Plato PA. In: Brueggeman P, Pollock NW, eds. Diving for Science 2008. Proceedings of the American Academy of Underwater Sciences 27th Symposium.
- The Complement System and Diving. Dr. Ernest Campbell FACS
- New Methods to Avoid Decompression Sickness. K Mogentale. ScientificAmerican.com 2012
- Microbubble Damage to the Blood-Brain Barrier: Relevance to Decompression Sickness. Hills BA, James PB. Undersea Biomed Res. 1991 Mar;18(2):111-6.
- Gene-environment mismatch in decompression sickness and air embolism. Alcock J, Brainard AH. Med Hypotheses. 2010 Aug;75(2):199-203. doi: 10.1016/j.mehy.2010.02.022. Epub 2010 Mar 19.
- Central fatigue: the serotonin hypothesis and beyond” Meeusen R1, Watson P, Hasegawa H, Roelands B, Piacentini MF. Sports Med. 2006;36(10):881-909.
- Evidence For Cerebral Perfusion Deficits in 28 Cases Of Dysbarism. Adkisson GH, Macleod MA, Hodgson M, et al. Lancet 2:119-121, 1989
- Microbubble Damage to the Blood-Brain Barrier: Relevance to Decompression Sickness‘. Hills B.A., James P.B. Undersea Biomedical Research. 1991, 18, 2:111-116
- Impact of Bubble Size in a Rat Model of Cerebral Air Microembolization. Juenemann et al. Journal of Cardiothoracic Surgery 2013, 8:198
Become A Shearwater Power User! (eBook)
An Illustrated Guide to Gradient Factors and Advanced Shearwater Computer Settings for safe scuba diving.
42 Pages. Printable PDF format. Fully Illustrated. $9
Become A Shearwater Power User eBook
As featured on the DiveTalk Podcast
About The Author
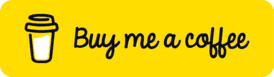
Andy Davis is a RAID, PADI TecRec, ANDI, BSAC, and SSI-qualified independent technical diving instructor who specializes in teaching sidemount, trimix, and advanced wreck diving courses.
Currently residing in Subic Bay, Philippines; he has amassed more than 10,000 open-circuit and CCR dives over three decades of challenging diving across the globe.
Andy has published numerous diving magazine articles and designed advanced certification courses for several dive training agencies, He regularly tests and reviews new dive gear for scuba equipment manufacturers. Andy is currently writing a series of advanced diving books and creating a range of tech diving clothing and accessories.
Prior to becoming a professional technical diving educator in 2006, Andy was a commissioned officer in the Royal Air Force and has served in Iraq, Afghanistan, Belize, and Cyprus.
In 2023, Andy was named in the “Who’s Who of Sidemount” list by GUE InDepth Magazine.
Purchase my exclusive diving ebooks!
Originally posted 2018-03-07 23:56:46.