Clearing Up The Confusion About Deep Stops
Deep Stops Theory by Erik C. Baker
The old adage, “an ounce of prevention is worth a pound of cure”, is certainly applicable to the various symptoms of decompression sickness (DCS).
The best treatment of all for these maladies is to complete a sufficient decompression profile in the first place. Technical divers have observed that many ailments can be avoided by including deep stops in their profiles.
A closer examination of the decompression model reveals that this practice serves to reduce or eliminate excessive overpressure gradients.
Knowing this, the model can be modified to provide precise control of gradients and stops can be calculated within the decompression zone to the depth of the “deepest possible decompression stop.
Many technical divers have observed that they feel fatigue, malaise, or drowsiness after completing certain types of decompression dives.
Bounce Dives and Deep Stops
The “bounce dive,” which is characterized as being relatively deep with a short bottom time, often produces such symptoms. Conventional implementation of the dissolved gas decompression model for this kind of dive will generate a first stop in the decompression profile that is much shallower than the bottom depth.
Several divers have reported that if they add some “deep stops” in their profiles, i.e., deeper than what the conventional calculation calls for, then post-dive symptoms are dramatically reduced or eliminated. Issues of confusion and controversy among technical divers are the questions of how deep these “deep stops” should be and how many such stops should be performed.
The empirical observations of divers have led to the development of arbitrary methods for introducing deep stops. Many of these methods involve individual judgment and discretion rather than having a basis in the decompression calculations.
Analysis of complete decompression profiles that utilize arbitrary deep stops reveals that there are potential problems. These include stops that are made too deep and inadequate extension of decompression time at the shallow stops to compensate for the increased gas loading caused by the deep stops.
CONVENTIONAL DECOMPRESION CALCULATION
In decompression application and theory, there is a trade-off between sufficient decompression (no symptoms of DCS) and economic decompression (minimum amount of time, gas supplies, exposure, etc.).
Robert D. Workman and Albert A. Bühlmann
Conventional dissolved gas algorithms, such as those developed by Robert D. Workman and Albert A. Bühlmann, seek to optimize the decompression by allowing the diver to ascend to the shallowest depth or “ceiling” based on the ascent limiting M-values for the hypothetical “tissue” compartments.
Become A Shearwater Power User! (eBook)
An Illustrated Guide to Gradient Factors and Advanced Shearwater Computer Settings for safe scuba diving.
42 Pages. Printable PDF format. Fully Illustrated. $9
Become A Shearwater Power User eBook
As featured on the DiveTalk Podcast
The economics of this are two-fold: inert gas elimination in the faster compartments is accelerated while inert gas uptake in the slower compartments is minimized during decompression. In practice, divers have traditionally been instructed to “get off the bottom” and ascend to the first stop in a timely manner.
For a typical bounce dive, the conventional calculation will permit a relatively long ascent from the bottom to the first stop. In this scenario, the inert gas loadings in the fastest compartments can be at or near saturation at the bottom depth while the slowest compartments are only partially loaded.
This means that the fastest compartments will control the initial ascent since their inert gas loadings will be closer to M-values well before the slower compartments. The first stop is determined when the inert gas loading in the leading compartment is equal to or near its M-value.
BUBBLES AND GRADIENTS: Robert D. Workman
When the concept of M-values was first presented in 1965 by decompression researcher Robert D. Workman, an assumption was made that inert gas would not come out of solution as bubbles in a diver’s tissues until an M-value was exceeded.
This theory was somewhat controversial at the time, however it was recognized that future technology would be able to give better information about the presence and behavior of bubbles in diver’s bodies.
Workman acknowledged that “ultrasonic methods of bubble detection in vivo and in vitro are being explored to permit better definition of decompression adequacy, but this is still in its early stages.”
Doppler ultrasound technology
Since then, Doppler ultrasound technology was developed and it has been used extensively in decompression research around the world. This research has shown that bubbles are present in the body’s circulation during and after many kinds of dives, including those with no symptoms of DCS.
In other words, a diver does not have to exceed an M-value in order to generate bubbles. This fact has been acknowledged in decompression science, but the mechanisms for bubble formation and growth in the human body are neither well understood nor precisely defined.
The laws of physics and many bubble models predict that greater numbers and sizes of bubbles can be expected with increasing overpressure gradients.
In the dissolved gas model, this means that more bubbling can be expected as the compartment inert gas loadings plot farther above the ambient pressure line on the pressure graph.
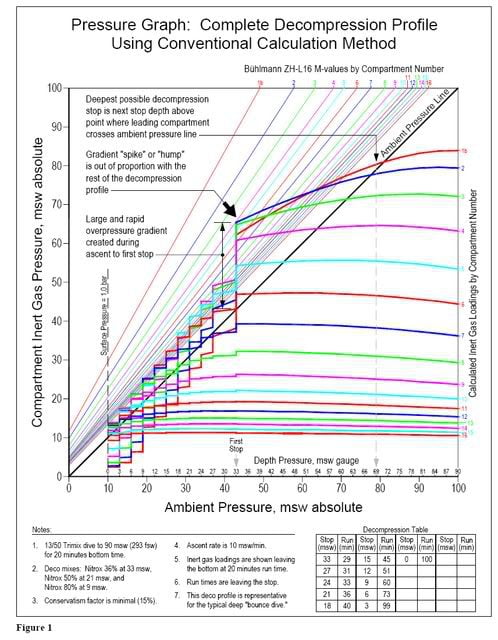
ILLUSTRATING THE PROBLEM
The pressure graph in Figure 1 shows a complete decompression profile calculated by the conventional method. In this profile, the fastest compartments have the greatest gas loadings during the initial ascent and are leading.
The M-values for these fast compartments permit large overpressure gradients relative to the slower compartments.
Consequently, a large and rapid overpressure gradient is created during the ascent to the first stop. This is out of proportion with the smaller overpressure gradients permitted during the rest of the decompression profile when slower compartments are controlling.
Presumably, many bubbles could be generated during the initial ascent to the first stop. In this case, the calculated gradient is 22.4 meters of seawater (msw) . 73 feet of seawater (fsw) . 2.2 atmospheres.
For comparison, when a can of soda is opened, the pressure gradient between the dissolved carbon dioxide and the air is in the range of 3.1 to 3.4 atmospheres.
Although an M-value is not exceeded in the decompression profile of Figure 1, a diver could experience symptoms of fatigue, malaise, or drowsiness after this dive.
Explanations for this include theories of bubble migration within the body and delayed off-gassing caused by accumulation of bubbles in the pulmonary capillaries.
In any case, it is possible to associate a cause and effect relationship between large overpressure gradients during the dive and post-dive symptoms.
Mild or vague symptoms such as fatigue and malaise, which normally do not receive medical treatment, may fall within a category of decompression stress, a lesser variant of DCS.
SOLUTIONS TO THE PROBLEM
Large and/or rapid overpressure gradients in a decompression profile presumably create more bubbling which leads to decompression stress or DCS.
Limiting Overpressure Gradients
The obvious solution to this problem is to limit the magnitude of overpressure gradients. Information within the dissolved gas decompression model can be used to address the issue.
First, there is a limit to how deep a “deep stop” can be. The leading compartment inert gas loading associated with a “decompression stop” should not be below the decompression zone.
In general, an overpressure gradient of some magnitude is required for efficient off-gassing. It is also important to minimize the extent of on-gassing in the slower compartments during the decompression.
Within the context of the dissolved gas model, the “deepest possible decompression stop” for a given profile can be defined as the next standard stop depth above the point where the gas loading for the leading compartment crosses the ambient pressure line (see Figures 1 thru 3).
The deepest possible stop depth is easily calculated in a decompression program and it will vary depending on the ascent rate from the bottom and the gas mix being used.
A decompression profile does not necessarily need to have a first stop at the deepest possible stop depth. This depth simply represents the point where at least one compartment will be in the decompression zone.
For many decompression profiles, stops that start a few standard stop depths above the deepest possible stop should be adequate to control excessive overpressure gradients. However, the deepest possible stop depth is valuable information for the diver as it represents the beginning of the decompression zone.
When reaching this point during the ascent from the bottom, the diver should slow the ascent to the decompression zone rate of 10 msw/min (30 fsw/min) or less. This practice will help to reduce rapid changes in overpressure gradients which presumably promote bubbling.
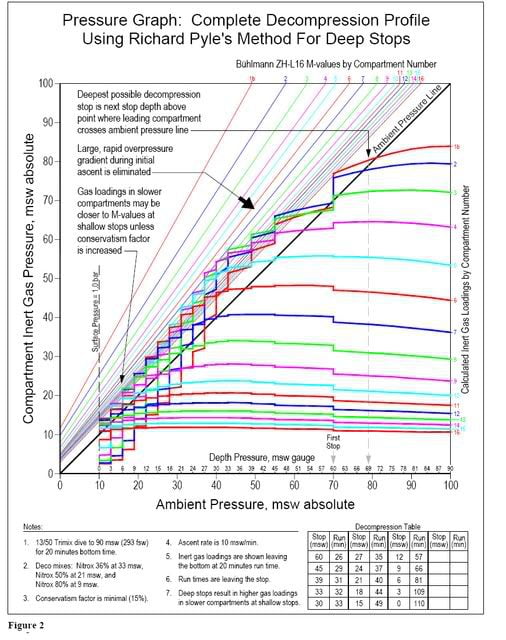
Richard L. Pyle Stops
Next, there is the issue of introducing deep stops. One empirically-derived method for deep stops was published by diver and marine biologist Richard L. Pyle. It is used in conjunction with a desktop decompression program with multi-level capabilities.
A complete decompression profile using Richard Pyle’s method for deep stops is shown on the pressure graph in Figure 2. The graph indicates that this method is effective in reducing or eliminating excessive overpressure gradients when compared to the conventionally calculated profile.
There are, however, potential difficulties with this approach. Depending on the decompression program being used and its method for conservatism, the gas loadings in the slower compartments may be closer to the M-values at the shallow stops due to the increased uptake caused by the deep stops.
The program will compensate for the deep stops, but unless the conservatism factor is increased, it may not provide the same margin of safety at the shallow stops as when running a conventional profile.
A good way to evaluate this is to calculate the maximum Percent M-values and Percent M-Value Gradients across all compartments at each stop.
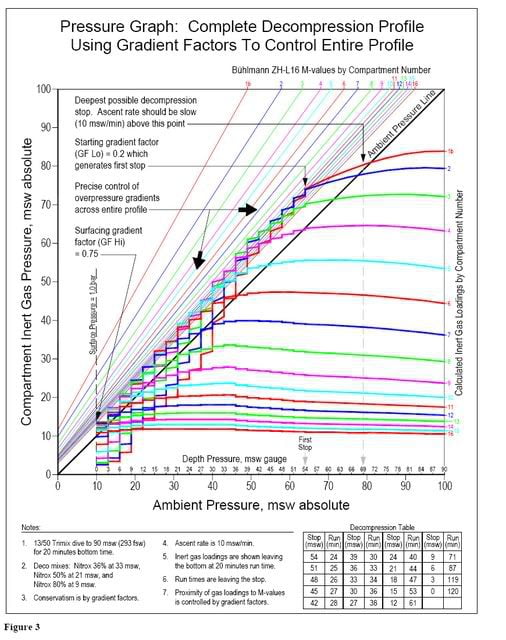
The pressure graph in Figure 3 shows a complete decompression profile calculated using Gradient Factors to control the overpressure gradients across the entire profile.
Gradient Factors provide a consistent approach to conservatism in decompression calculations. They can be used to generate deep stops within the decompression zone, control overpressure gradients, and ensure a fixed margin of safety from the M-values across the entire decompression profile.
A Gradient Factor is simply a decimal fraction or percentage of the M-Value Gradient
The addition of deep stops in a profile will generally increase the time required at the shallow stops as well as the overall decompression time.
However, if truly “sufficient decompression” is the result, then the concept of “economic decompression” is not really compromised.
The pressure graph is an excellent tool for divers to evaluate decompression profiles. Even a quick review can identify potential problem areas such as large overpressure gradients. Decompression modelers and programmers are encouraged to include this feature in their programs.
Finally, the example decompression profiles used for the pressure graphs in this article were calculated with minimal conservatism and are intended for comparison purposes only.
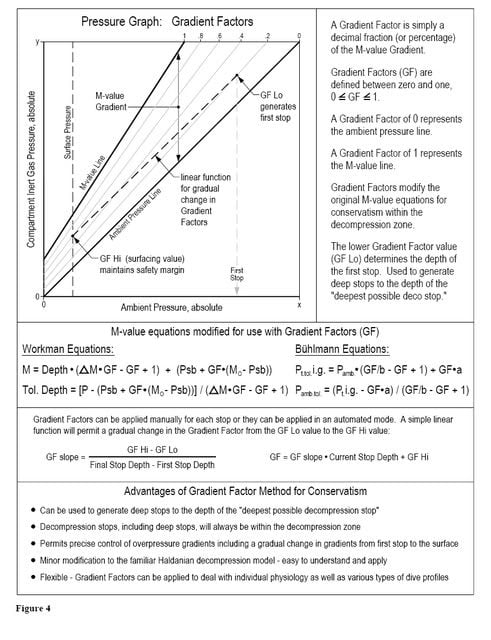
Decompression Deep Stops References
- Baker EC. 1998. Understanding M-values. Immersed. Vol. 3, No. 3.
- Bennett PB, Elliott DH, eds. 1993. The Physiology and Medicine of Diving. London: WB Saunders.
- Bühlmann, AA. 1984. Decompression-Decompression Sickness. Berlin: Springer-Verlag.Bühlmann, AA. 1995. Tauchmedizin. Berlin: Springer-Verlag.
- Hamilton RW, Rogers RE, Powell MR, Vann RD. 1994. Development and validation of no-stop decompression procedures for recreational diving: The DSAT Recreational Dive Planner. Santa Ana, CA: Diving Science and Technology Corp.
- Pyle RL. 1996. The importance of deep safety stops: Rethinking ascent patterns from decompression dives. DeepTech. 5:64; Cave Diving Group Newsletter. 121:2-5.
- Schreiner HR. 1968. Safe ascent after deep dives. Rev. Subaquat. Physiol. Hyperbar. Med. 1:28-37.
- Schreiner HR, Kelley PL. 1971. A pragmatic view of decompression. In: Lambertsen CJ, ed. Underwater Physiology IV. New York: Academic Press.
- Wienke BR. 1991. Basic decompression theory and application. Flagstaff, AZ: Best.
- Wienke BR. 1994. Basic diving physics and applications. Flagstaff, AZ: Best.
- Workman RD. 1965. Calculation of decompression schedules for nitrogen-oxygen and helium-oxygen dives. Research Report 6-65. Washington: Navy Experimental Diving Unit.
Become A Shearwater Power User! (eBook)
An Illustrated Guide to Gradient Factors and Advanced Shearwater Computer Settings for safe scuba diving.
42 Pages. Printable PDF format. Fully Illustrated. $9
Become A Shearwater Power User eBook
As featured on the DiveTalk Podcast